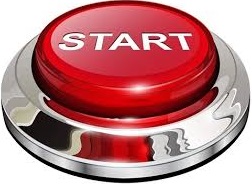
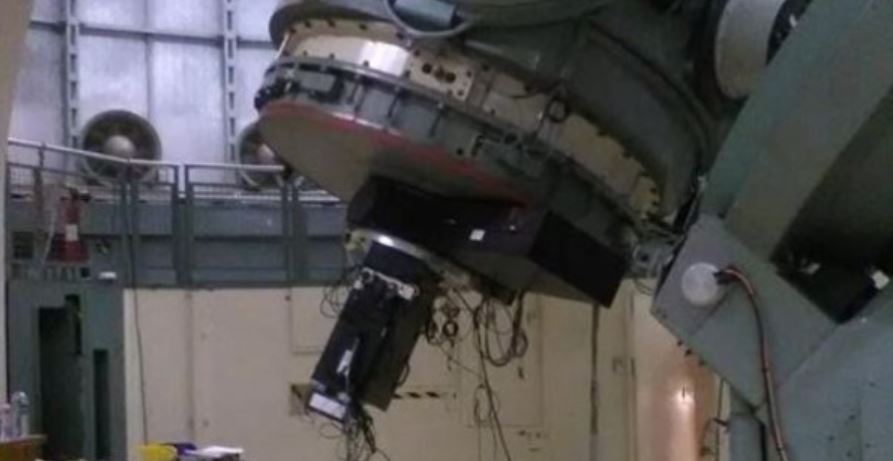
To lower the cost of light source (one of the most expensive items), the industry-grade laser was proposed to replace the scientific grade laser, which has shown a decent performance in SMLM 8, 9. To meet these technical requirements and achieve the best performance, it is a common belief that the high-end optical and optoelectronic components have to be used, including high-power single-mode laser, high-end objectives, nano-positioning mechanical system and highly sensitive cameras, which easily drive the total cost to exceed $100,000. The key technical requirement for SMLM includes (1) a powerful laser to photo-bleach most fluorophores and activate only a small portion of sparsely distributed single fluorophores (2) high numerical aperture (NA) objective lens to efficiently collect the limited number of photons emitted by single molecule and (3) scientific cameras with high quantum efficiency and low noise to record the image from individual fluorescent emitters at a high signal to noise ratio (SNR). Its high cost limits its widespread use as a routine microscopy system as conventional fluorescence microscope. However, despite that SMLM has been commercialized for almost a decade, it remains a high-end microscopy instrument only available in the imaging facilities at major academic institutions and a small number of laboratories.
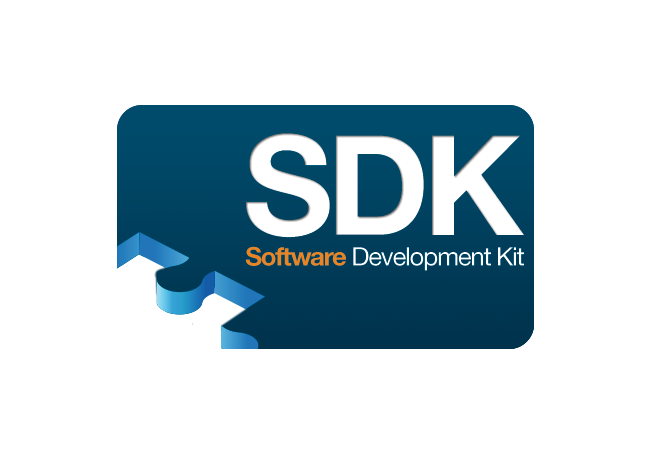
In particular, single molecule localization microscopy (SMLM), which provides impressive spatial resolution down to 20 nm with relatively simple hardware compared to SIM and STED, has a great potential as a common laboratory tool for biomedical research. These super-resolution techniques have improved the spatial resolution by approximately an order of magnitude. These super-resolution techniques can generally be divided into two categories: the technique based on the nonlinear effects of the fluorescence that sharpens the point spread function (PSF) using modulated light, including stimulated emission depletion (STED) 1 and structured-illumination microscopy (SIM) 2, 3 and the technique based on localization of sparsely excited single fluorescent emitters, including (fluorescence) photo-activated localization microscopy 4, 5 and (direct) stochastic optical reconstruction microscopy 6, 7. Until the recent decade, a number of super-resolution imaging techniques have been developed to break the diffraction barrier. However, due to the diffraction-limited spatial resolution, conventional fluorescence microscopy cannot visualize biological structures smaller than ~200 nm. In the past few decades, fluorescence microscopy has significantly expanded our ability to study biological processes at the cellular and subcellular level.
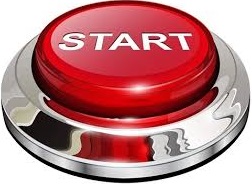